Chapter 4 Phylogenesis and Evolution
In the middle of the model, we see the biological aspect “phylogenesis” indicated with the red box in Figure 4.1, which is our focus in this chapter.
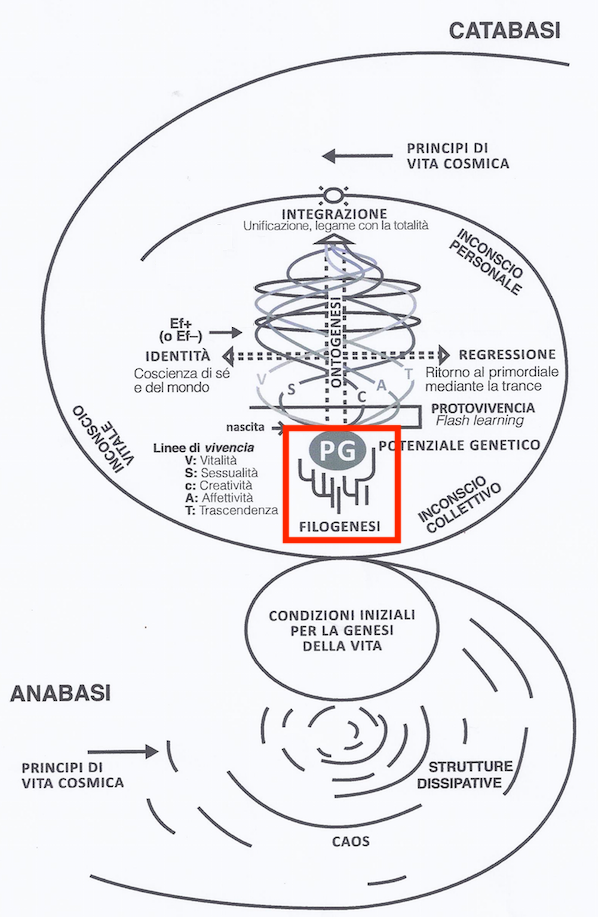
Figure 4.1: Model of Biodanza and Phylogenesis
Phylogenesis is the biological process or evolutionary mechanism by which a species or a group of species appears. This concept was very important for Rolando Toro, as it shows how related all living organisms are and how natural it is that their lives are all structured according to the same principles.
For Rolando, evolution went further than biological evolution. Indeed, according to him, life, in essence, consists of two important processes: Anabasis and Catabasis, from which all structures in the universe did arise. Anabasis is the integration from chaos to self-organising systems while Catabasis refers to the disintegration of such systems, which however, immediately carries the seed for novel creation. With the evolution of the universe more complex structures did arise. This, however, does not imply that evolution is directional, i.e. going from the “simple” to the “higher or more complex”, a confusion that is widespread. Instead, we can see these two organizing principles as a creative process, that leads to the generation of novel structures, some are more simple, while others are more complex; and all of them have their own rhythm and unique cosmic dance. So, in essence evolution gives rise to a wealth of diversity.
According to Rolando all structures in the universe are shaped by the same principles: attraction and repulsion. However, the expression of these principles is differentiated each time a novel structure emerges and also varies in its complexity. Indeed, attraction and repulsion can be observed from a simple atom up to the level of galaxies; and in biological lifeforms attraction and repulsion further differentiated into
- positive and negative phototaxis9, geotaxis10 and chemotaxis11,
- reflexes, simple responses to stimuli
- instincts,
- emotions, up to
- feelings.
All of these are key for us to remain in homeostasis, i.e. the self-regulating process by which an organism tends to maintain its stability and internal equilibrium in its ever changing environment, while adjusting to conditions that are best for our survival.
Interestingly, Margulis (1999) mentioned that “metabolizing ancient bacteria were so effective at remaking themselves (…) that the insides of our bodies today are chemically more akin to the external environment of the early Earth, in which life originated, than they are like our present oxygen-rich world.” Indeed, “life has been, since inception and with no discontinuity, chemically connected to its past” and Margulis (1999) continues that “the metabolic memory of modern cells most likely predates most ancient rocks”. So, memory can be seen as an intrinsic property of biological systems and their metabolic arsenal is a reflection of the environments it has been exposed to during its evolutionary trajectory.
According to Rolando, the differentiation of autonomy is another important aspect of evolution. Indeed autonomy gives an organism the capacity to react, to maintain homeostasis, and thus the ability to integrate with its external environment without losing its identity. The differentiation of autonomy is reflected in the differentiation of an organism’s (a) sensory-motor, (b) immune, and, (c) cognitive and communicative arsenal, which gives it (a) the plasticity to adapt, (b) the capacity to preserve its biological integrity by recognizing what is endogenous and foreign, and, (c) the ability to recognise and interact with other members of its own species, respectively. These three important functions were already provided by some distinct sets of proteins in bacteria and evolved towards distinct and complex systems in plants and animals.
Hence, it is extremely valuable to study what life has in common, so as to better understand ourselves, and, to feel (re)connected and united with life and the entire universe.
We start this chapter with two sections that are more technical and focus on the concepts related to Phylogenesis and Evolution introduced in the reader of the Biodanza teacher training “Module IV: Biological aspects of Biodanza”. We conclude this chapter with a more philosophical section on evolution, humanity and Biodanza.
4.1 Phylogenesis
All species evolved from the same ancestral population of cells. This is also referred to as the same Last Universal Common Ancestor (LUCA). In the tree of life the evolutionary relations are summarized between different organisms (and groups of organisms). All living beings eventually can be traced back to the last universal common ancestor who is located at the root of the tree, see Figure 4.2.
Figure 4.2: The tree of life is one of the most important organizing principles in biology. It shows the evolutionary relationships among different organisms and that all living beings eventually can be traced back to the last universal common ancestor who is located at the root of the tree (Source: wikipedia)
Phylogenesis is the process of the origin of all species from the tree of life from LUCA.
Rolando Toro refers to the origin of species and adaptation to the environment as evolutionary differentiation and he considers the genetic information of a species as a record of the environments and evolutionary development that it underwent up to this point.
4.1.1 Timescale
4.5 BYA | 4.3 BYA | 3.8 BYA | 3.5 BYA | 540 MYA | 520 MYA |
---|---|---|---|---|---|
(Source: naturedocumetaries.org)
At its origin about 4.5 Billion Years Ago (BYA) the Earth was black, a hot basalt rock and dust in a cold vacuum. As the Earth cooled down she became Grey Earth (4.3 BYA) as she was mainly covered by granite and rocks. Another 500 million years later she was covered with liquid water: Blue Earth (3.8 BYA).
In only 300 million years she radically changed into Red Earth (3.5 BYA) due to life. Indeed, cyanobacteria emerged that can do photosynthesis and produce oxygen, a very reactive molecule. This led all free iron in the ocean to precipitate as iron oxide or rust (red). There was an explosion of the number of minerals, they went from approximately 250 to more than 5000 mineral species. Oxygen also caused a mass extinction because only few organisms could cope with its highly reactive nature. The coming three billion years life on Earth stayed relatively similar.
Around 540 MYA Earth was struck by a large ice age, White Earth. Again leading to a mass extinction due to the cold. However, volcanic activity came to the rescue by producing greenhouse gasses and an atmosphere that could retain more heat.
In less than 20 million years (MY) Earth radically changed again and turned into Green Earth (520 MYA). There was an explosion of life, and life suddenly evolved from being mainly unicellular to complex multicellular forms.
4.2 Evolution
How does the evolution of LUCA to all species of the tree of life occurred?
Lamarck was one of the first proponents of biological evolution and proposed the theory that organisms altered their behavior in response to environmental change and that their changed behavior, in turn, modified their organs, and their offspring inherited those “improved” structures. A theory that has been abandoned in favor of Darwin and Wallace’s theory of evolution by natural selection. For them, the mechanisms of reproductive heritability and the origin of new traits, however, remained a mystery. Upon the rediscovery of the work of Mendel natural selection and population genetics were combined in the neo-darwinian theory or the synthetic evolution theory. Key principles in this theory are variability and selection.
The groundbreaking work of Margulis, however, shed new light on evolution. According to her theory, symbiosis is another important driver of evolution. Symbiosis is a close, prolonged association between two or more different biological species where the involved species all benefit from their interaction. Particularly, her theory of sequential endosymbiosis, where different types of bacteria fused together to form larger and more complex cells, can explain the sudden explosion of life forms that was observed from 540 MYA - 520 MYA.
In this section we first focus on the principles of variation and selection. We will then continue with endosymbiosis and symbiogenesis. We conclude this section with elaborating on the concept of teleonomy.
4.2.1 Variability and Selection
Variation occurs, among others, through errors in the copying of DNA for cell division of prokaryotic cells and gamete12 production for eukaryotic cells (see Figure 4.3).
Figure 4.3: Copying of DNA by DNA-polymerase. DNA polymerase extends a DNA strand by incorporating a complementary nucleoside-tri-phosphate and splitting two of the three phosphate groups. Note, again the link between energy and information. Errors are often corrected by proofreading. However, some of the errors remain.
The error margin of DNA replication is 1 error per billion base pairs that are copied, which can lead to point mutations. Note, that we humans have a genome size of about 6.4 billion base pairs.
Other sources of variability are
- Insertions or deletions, i.e. base pairs that are added or removed, respectively.
- Recombination, reshuffling of genetic traits, e.g. during sexual reproduction
Most mutations are neutral, i.e. the mutated codon encodes for the same amino acid. Neutral mutations can be used as a molecular/genetic clock to tell how far apart two species are in an evolutionary sense.
But, mutations are not always neutral! For instance, sickle cell anemia is caused by one point mutation in hemoglobin (see Figure 4.4 and 4.5). A T (thymine base) is mutated to an A (adenine base), which results in a codon that encodes for the amino acid valine instead of glutamic acid. This causes dramatic changes to the 3D structure of the hemoglobin protein and causes a deformation of the red blood cells from a round to sickle shape, which leads to anemia.
Why does this mutation remain? It mainly occurs in Africa, where the mutation for sickle cell anemia is selected because it makes affected individuals resistant against malaria, which gives them a evolutionary competitive advantage despite their anemia. However, offspring who inherit the mutation of both parents are not viable. Therefore, the regular variant of hemoglobin also remains.
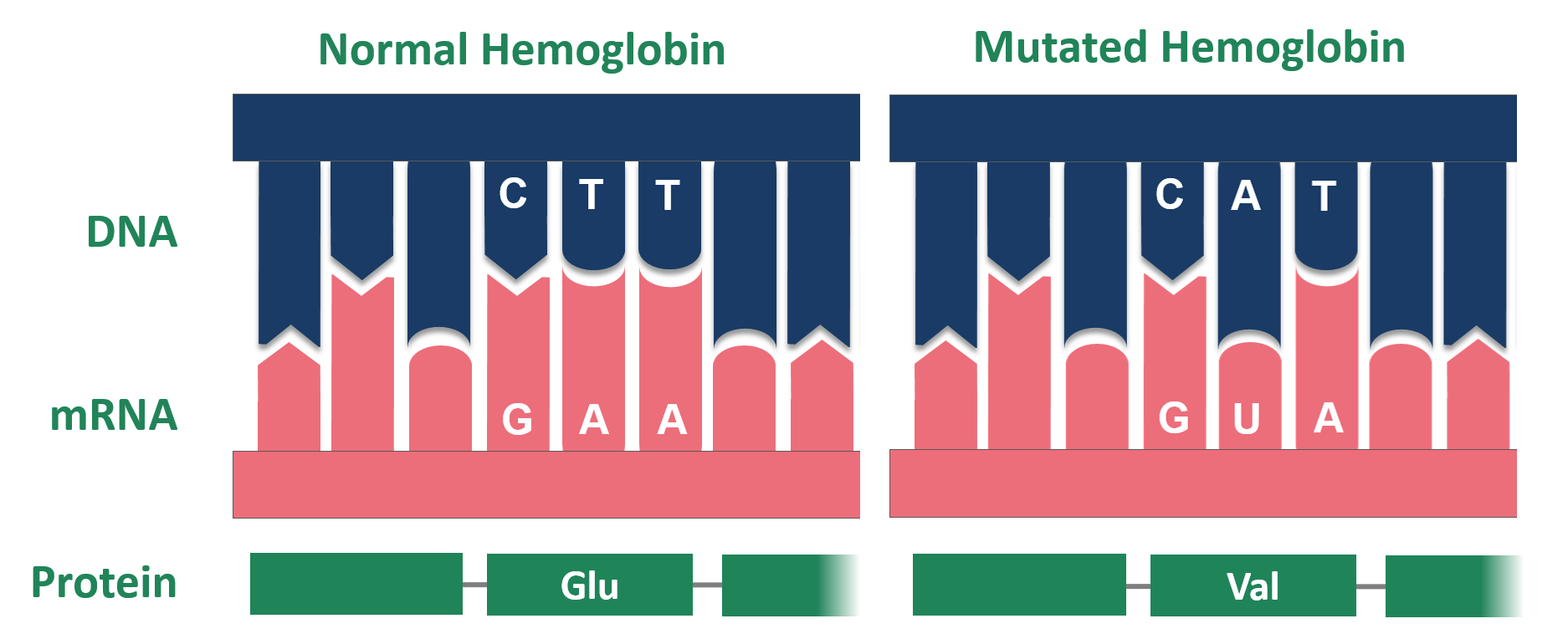
Figure 4.4: Mutation of Hemoglobin in patients with sickle cell anemia (Source: Wikipedia)
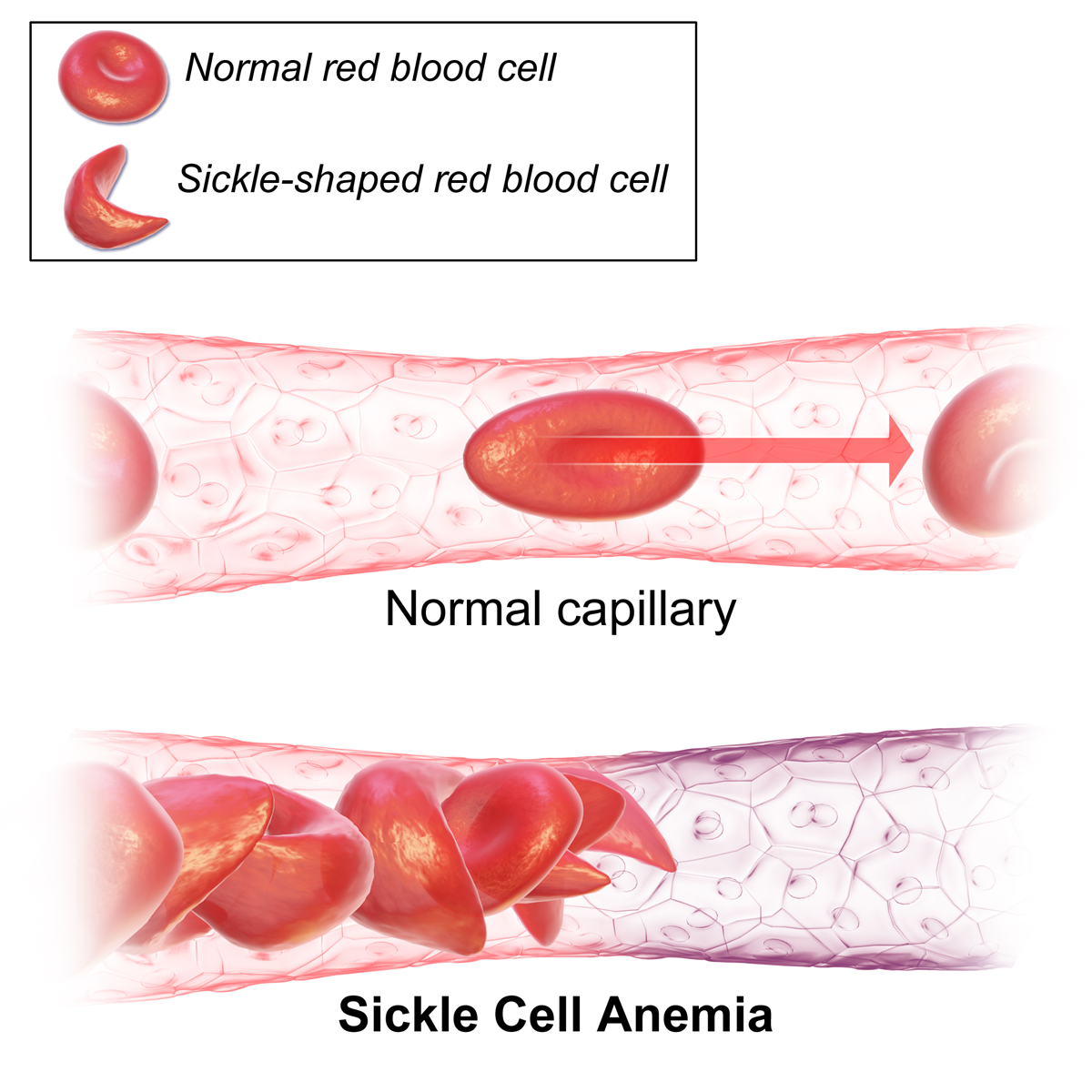
Figure 4.5: Deformed red blood cells in patients with sickle cell anemia (Source: Wikipedia)
Hence, evolution is a natural process that is driven by two opposing forces: variation and selection.
Variation occurs by spontaneous copy errors in genetic code or mutations, among others.
Selection occurs upon ecofactors: Is a mutation beneficial or harmful for a particular organism in its specific environment? The odds on fixation of the mutation thus depends on the reproductive success.
The process of genetic variation and selection can eventually lead to evolution of new species upon many generations.
4.2.2 Genetic Drift
Another important process for the origin of species is genetic drift. This are random fluctuations of alleles, which are particular sequence variants of a gene. Genetic drift is particularly strong in small populations. As opposed to selection it is not adaptive.
New species will thus originate more quickly when a small fraction of the population gets isolated in a new environment.
4.2.3 Horizontal Gene Transfer
Horizontal gene transfer, which is non-sexual transfer of genetic information between two distinct organisms, is also important for the evolutionary process.
This is very common between Prokaryota, i.e. eubacteria and archaea bacteria, think on the super bugs in hospitals that acquired resistance to multiple antibiotics. It also occurs between Eukaryota. Mainly in protists, which are unicellular organisms with a nucleus. As well as between Prokaryota on the one hand and Eukaryota on the other hand.
4.2.4 Endosymbiosis
Niles Eldredge and Stephen Jay Gould have shown that the fossil record indicates that evolution happens in bursts: it is slow most of the time until suddenly rapid changes occur over a brief time (Margulis 1999). According to Lynn Margulis this can be explained by endosymbiosis, where one species starts to live within another species, which is a source of evolutionary novelty that can give rise to an explosion of novel life forms.
There exist two archetypes of cells:
- Prokaryota, bacteria and archae-bacteria, are unicellular. They have simple cells of a size of 0.1 to 5.0 \(\mu m\) with DNA that is lying freely in the cell cytosol (the fluid in the cell), see Figure 4.6 and
Figure 4.6: Diagram of a typical prokaryotic cell. The cell is simple, the DNA is lying free in the cell (Source: Ali Zifan, Wikipedia)
- Eukaryota, protists, fungi, plants and animals, are unicellular or multicellular. Their cells are larger and more complex. Their size ranges from 10-100 \(\mu m\). They have a variety of internal membrane-bound structures, called organelles, and a cytoskeleton, which play an important role in defining the cell’s organization and shape. Eukaryotic DNA is stored in chromosomes. A chromosome is a long DNA molecule that is stored in a compact form. A chromosome contains a part or all of the genetic material of an organism. Human cells have 46 chromosomes, i.e. 23 chromosome pairs. Each pair consists of one copy from our biological mother and one from our biological father. The chromosomes are located in the cell nucleus, which is the organelle maintaining the integrity of chromosomes and thus of our genes, and plays an important role in the regulation of gene expression and of the activities of the cell. See Figures 4.7 and 4.8.
Figure 4.7: Diagram of a typical animal cell. Animal cells are eukaryotic cells. They are typically much larger than those of prokaryotas. They have a variety of internal membrane-bound structures, called organelles, and a cytoskeleton, which play an important role in defining the cell’s organization and shape. Eukaryotic DNA is divided into chromosomes, that are located in the cell nucleus, which is the organelle maintaining the integrity of genes, and plays an important role in the regulation of gene expression and of the activities of the cell. (Source: Mariana Ruiz Villarreal, Wikipedia)
Figure 4.8: Diagram of a typical plant cell. Plant cells are eukaryotic cells. They are typically much larger than those of prokaryotas. They have a variety of internal membrane-bound structures, called organelles, and a cytoskeleton, which play an important role in defining the cell’s organization and shape. Eukaryotic DNA is divided into chromosomes, that are located in the cell nucleus, which is the organelle maintaining the integrity of genes, and plays an important role in the regulation of gene expression and of the activities of the cell. Plant cells also have a cell wall and chloroplasts that can perform photosynthesis. (Source: Mariana Ruiz Villarreal, Wikipedia)
From 3.5 BYA - 540 MYA we find mainly prokaryotic and some simple eukaryotic organisms in fossils and life was mainly unicellular.
Margulis sequential endosymbiosis theory (SET) postulates that eukaryotic cells are generated by endosymbiosis, i.e. a symbiotic relationship where one organism started to live inside the other, which is beneficial for both organisms. The process of sequential endosymbiosis that led to eukaryotic cells is displayed in Figure 4.9.
Figure 4.9: Genesis of eukaryotic cells (Source: wikipedia)
First an anaerobic13 prokaryotic cell was assumed to have grown and to have developed membrane systems inside the cell, which gave rise to the formation of the nucleus14. At a certain point of time this large prokaryotic cell with internal membrane systems must have ingested an aerobic15 proteobacterium, which managed to avoid digestion. The two cells started with an endosymbiotic relation.
The ingested proteobacterium provided the host cell with additional energy through the synthesis of ATP by metabolizing carbohydrates and lipids using oxygen and the host cell fed the proteobacterium with these bio-molecules. This gave the endosymbiotic pair the ability to grow further in size and to thrive in the oxygen rich environment of planet Earth that was established during the Cambrian era that started 540 million years ago. The increase of oxygen is believed to be one of the major triggers that gave rise to the “biological big bang” that led to an explosion of novel species and the emergence of all large groups16 in the animal kingdom (He et al. 2019).
During their endosymbiotic evolution the proteobacterium gradually handed over many genes to the cell nucleus, and became under regulation of the nucleus. It eventually evolved to mitochondria, the organelles that are the energy factories of a cell. So, we inherit more from mother than father: we inherit our cell structure as well as our energy system, i.e. our mitochondria and their remaining DNA, from mother side through her egg cell.
For plant cells, a second endosymbiotic event must have occurred with eukaryotic cells and cyanobacteria. The ingested cyanobacteria has evolved in a similar way into chloroplasts, which give plants the ability for photosynthesis and led them become the most successful life form of the globe. Fossil evidence of land plants dates back to 485 MYA - 420 MYA, however, phylogenetic analysis also suggests an earlier origin in the Cambrian (Strother and Foster 2021).
Other key differences between Prokaryota and Eukaryota are in terms of their reproduction. For Prokaryota that only takes place by cell division. A mutation in the DNA is thus fixed in all daughter cells. While nearly all Eukaryota have a phase of sexual reproduction. They are diploid organisms, i.e. they have two copies of each gene, one from fathers’ and mothers’ side. This enables successive mutations to be made in one copy because another functional copy of the gene is available. Moreover during sexual reproduction recombination of chromosomes occurs, i.e. reshuffling of paternal and maternal genes, which leads to much more variation.
The Eukaryota further evolved in protists, which are unicellular organisms, fungae, plants and animals. Margulis SET theory also supports a more intuitive organisation of the tree of live into a two part (eukaryotic vs prokaryotic) five kingdom taxonomy that reflects evolutionary history, see Figure 4.10: Prokaryotic bacteria and archae-bacteria evolved in eukaryotic Protista that further evolved into Plants, Fungae and animals.
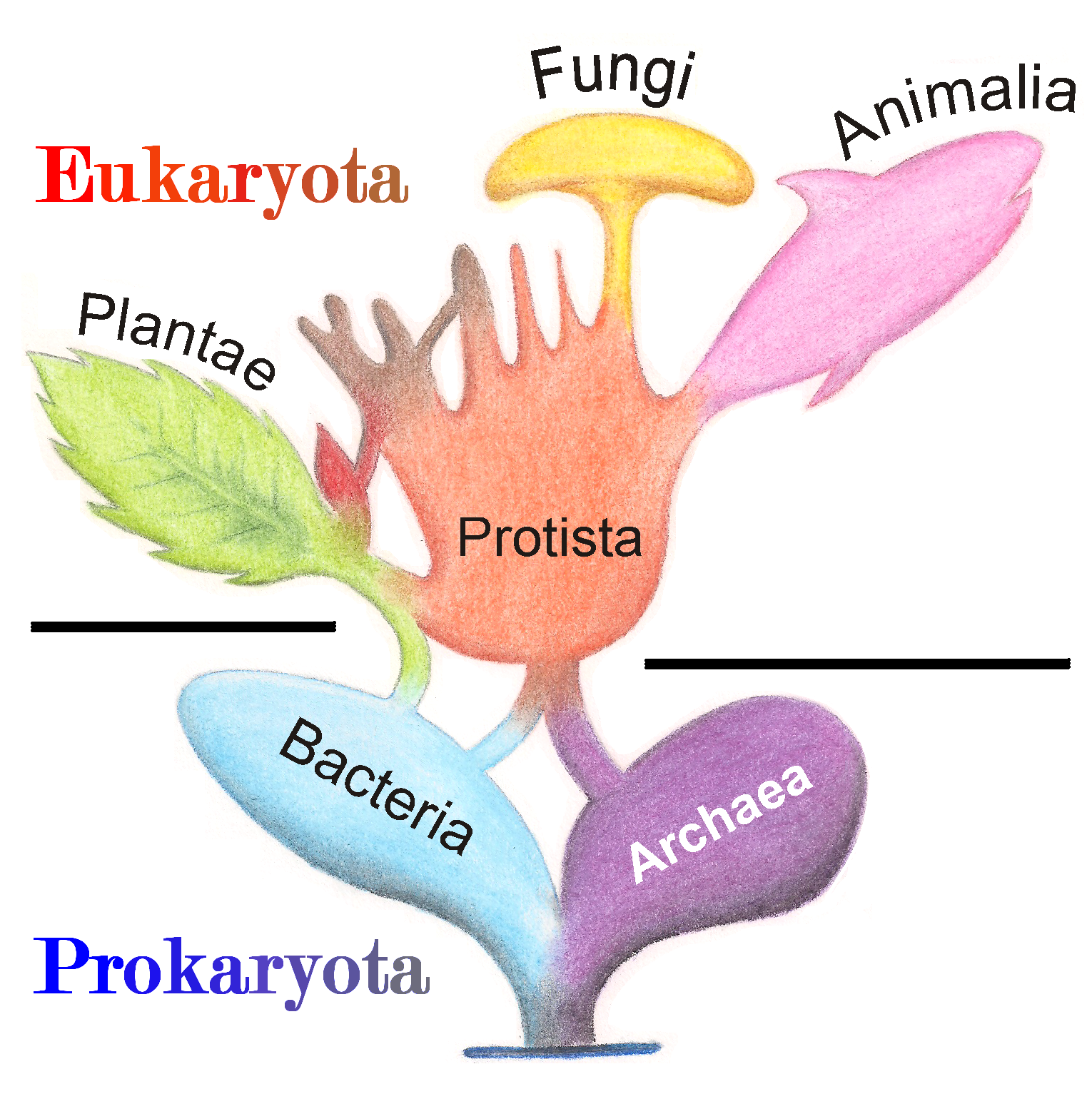
Figure 4.10: Two-part (eukaryotic vs prokaryotic) five kingdom taxonomy reflecting evolutionary history: prokaryotic bacteria and archae-bacteria evolved into eukaryotic Protista that further evolved into Plants, Fungae and animals (Source: wikipedia)
4.2.5 Symbiosis and Symbiogenesis
This section is largerly based on Margulis’ book “The symbiotic Planet” (Margulis 1999).
According to Lynn Margulis symbiosis, in general, is crucial to understand evolutionary novelty and the origin of species.
Symbiogenesis is an evolutionary term that refers to the origin of new tissues, organs, organisms and even species by the establishment of long-term and permanent symbiosis. As Lynn Margulis argued “all organisms large enough for us to see are composed of once-independent microbes that teamed up in larger wholes.” The fact that fungal, plant and animal cells originated by endosymbiosis is now widely accepted. However, symbiotic mergers continued through evolutionary history and were extremely important for life to colonize the entire globe.
Indeed, life evolved in the sea and symbiosis was the driver to colonize the hostile dry land. Symbiosis between fungi and cyanobacteria and green algae into lichens, for instance, has been key for the colonisation of the dry rocky surface and to turn rocks into fertile soil. The importance of lichens becomes clear from their biomass, which is even estimated to be larger than the biomass of all life in the ocean.
Once soil is available, plants can take over and again plant life crucially depend upon their symbiosis with fungi. Indeed, plant roots and fungi form mycorrhizae that are swollen symbiogenetic structures that provide the plant partner with mineral nutrients and the plant in turn delivers their fungal partner with sap or photosynthesized food. Their marriage turned plants into the most successful kingdom in terms of the mass of carbon that they fixate.
Similar examples hold for animals. Ruminants, e.g. cattle, sheep, antelopes, deer, giraffes, and their relatives, for instance would never have evolved without their symbiosis with cellulose degrading protists that enable them to digest grass. The same holds for us humans, the symbiosis with the microorganisms in our gut, our gut microbiome, is crucial for us to digest our food.
4.2.6 Teleonomy
Teleonomy is the quality of apparent purposefulness and of goal-directedness of structures and functions in living organisms brought about by evolutionary adaptation. So throughout evolutionary history, there was never a purpose to develop a particular trait other than the primitive goal to maintain and reproduce the species.
When complex organs and organisms originate it might seem as if there is a direction or purpose, but, that is not the case!
As Rosenberg and McShea (2008) nicely introduce in their book Phylosophy of Biology17: “For some, Darwin’s theory presents puzzles having to do with complexity, randomness, and directionality. They ask how complex functional designs can arise by a random process such as natural selection. For example, the evolution of a complex structure like a wing capable of sustained flight (as in a bird) from a fin (as in a primitive fish) might seem to be impossible, given the randomness of the process of natural selection and the enormous number of modifications necessary.”
“The first part of the answer is that natural selection is not random. It is a process that requires some randomness in its input. Variations arising are not targeted toward solving problems posed by the environment. But the output of natural selection is decidedly nonrandom, the differential survival and reproduction of the variants that are better adapted.”
“The second part of the answer is that natural selection can act cumulatively, and that is what makes complex adaptations possible. Selection first transformed a fin into a walking limb, strong enough to support a large animal on land, and then later transformed a walking limb into a wing, capable of producing sustained powered flight. Complexity is possible because later adaptations build on earlier adaptations. In other words, complex adaptations are not produced in big leaps but in smaller steps, each one of which is adaptive, and function can change from one step to the next. A wing is not a better fin or even a better leg. It is something entirely different, serving a different function. Looking only at the endpoints, the gap covered might seem impossibly large, and the reason is that natural selection to some extent covers its tracks. Looking at a fin or wing, the intermediate walking limb stage is not evident, at least not superficially evident. Though natural selection covers its tracks, a great deal of biology has been devoted to uncovering them. One of Darwin’s earliest arguments for natural selection was based on the close similarity in parts, their numbers, and spatial relations to one another (their homology) of the bones in fins, legs, and wings. Two hundred years later, molecular biologists can trace the genealogy of the bird’s wing back through the reptile’s leg to the fish’s fin in the similarities and differences of the gene sequences that control the development of each. They can show how the DNA sequence differences and similarities between the genes involved in limb development in birds, reptiles, and fish enable us to date their common ancestors and say something about how the homologies and the differences among the bones of their different limbs are due to differences in DNA sequences.”
![A small mutation can sometimes induce large phenotypic changes. Here, the mutation of the Antp gene is shown to lead to antenna-to-leg deformation in flies [@flyMut]. Panel A: Fly head with an antenna-to-leg deformation. Panel B: Fly head with a normal antenna formation.](figs/pone.0205905.g006_flyAntennaLegMutation2.png)
Figure 4.11: A small mutation can sometimes induce large phenotypic changes. Here, the mutation of the Antp gene is shown to lead to antenna-to-leg deformation in flies (Altamirano-Torres 2018). Panel A: Fly head with an antenna-to-leg deformation. Panel B: Fly head with a normal antenna formation.
Moreover, large phenotypic changes often involve very small genomic changes. Rosenberg and McShea (2008) continue that “In the fruit fly Drosophila, it is known that a small mutation in the right gene is all it takes to turn its antennae into a pair of legs. Increasingly, molecular biology is able to uncover the tracks evolution has hidden, so that adaptations begin to look expectable instead of miraculous. What about directionality? The notion of cumulative change might seem to suggest a kind of directedness to the process of adaptation, a drive toward greater complexity. In fact, however, it is an open question whether there is in evolution any preferred tendency for complexity to build up. What is clear, however, is that nothing in the current understanding of natural selection predicts a drive toward greater complexity. Increases occur, but in our fascination with them we tend to forget the frequent decreases. Winged animals become flightless, as in the evolution of penguins. Animals with walking limbs lose them when they return to the water, as in the evolution of whales (from a common ancestor with hippopotamuses!). Complexity is reversible, and selection is expected to favor decreases whenever opportunities for adaptive simplicity arise . . . which could be often!”
Hence, the origin of a species is the result of evolution, but, not the purpose of evolution. Evolution is thus adaptation with as sole goal maintenance and reproduction.
Moreover, from the distribution of the complexity of species in Figure 4.12, it is clear that the most abundant species in number always has been bacteria. The distribution of complexity at present, however, has a tail to the right. This mainly stems from the fact that there is lower bound of complexity of living organisms. Hence, evolution could not generate organisms with a complexity below this lower bound and it therefore only seems as if it slightly favors increasing complexity.
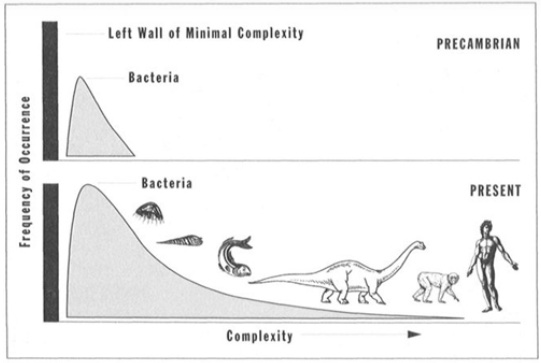
Figure 4.12: Distribution of complexity of species (Gould 1997)
Bar-On, Phillips, and Milo (2018) published the distribution of carbon mass fixated in different types of species in Figure 4.13, which also shows that there is no preference towards more complex organisms.
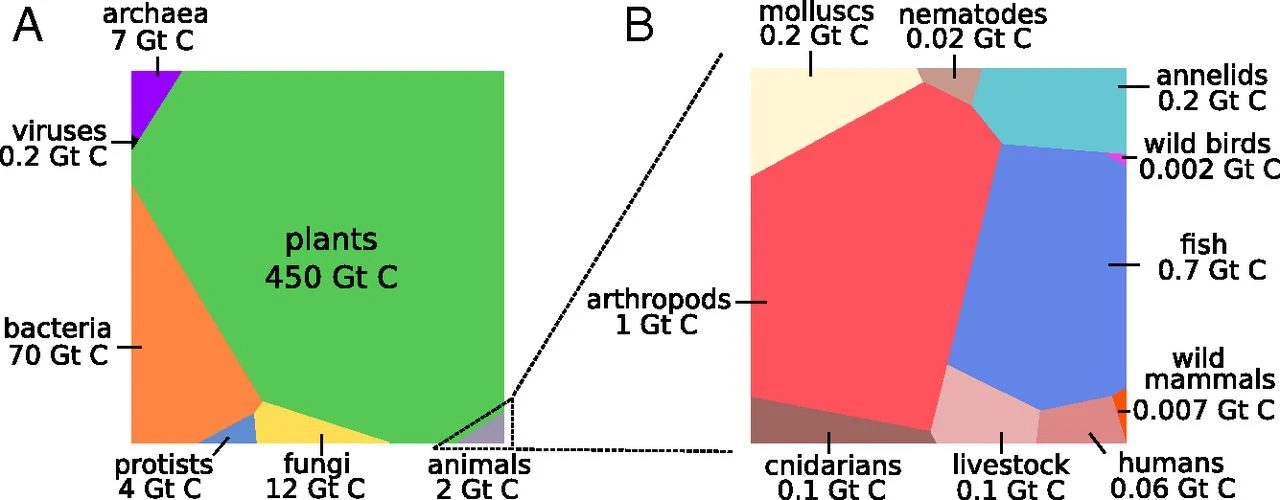
Figure 4.13: Mass in giga tons of carbon for different groups of species. (Bar-On, Phillips, and Milo 2018)
Note, that
Plants are the most successful group in terms of the mass of carbon that they fixate.
Bacteria clearly dominate the more complex animal kingdom.
Animals represent a relative small fraction. Among animals, cattle and humans are over represented.
Other compelling evidence that there is no direction to evolution stems from the number of bacterial cells in our body. Sender, Fuchs, and Milo (2016) showed that the ratio of the number of bacterial cells to the number of human cells is close to 1. A human with a weight of 70kg has \(\pm\) 38 trillion bacterial cells and 30 trillion humane cells. Note, that trillion is a thousand billion or 10\(^{12}\)!
Margulis’ take on this is nicely summarised in the following quote: “more complex multicellular organisms such as fungi, plants and animals can be seen as a massive colony of differentiated fused and mutating symbiotic microorganisms” (Margulis 1999).
So, all these arguments make clear that evolution has no direction. Instead, we can view evolution as a wonderful creative process that is generating novel life forms that are well adapted to their ever changing environment. So this insight on evolution invites us to deeply embrace the wealth of diversity, instead.
4.3 Evolution, Humanity and Biodanza
First there was
Chemical evolution: evolution of building blocks and chemistry of life.
Then, biological evolution of cells/organisms based on the selection of genetic information and function.
And finally, our species introduced cultural evolution that can bypass natural evolution using
- artificial selection: breeding of plants, pets, cattle, genetic manipulation, etc.
- Technology: that enables us for a fast adaptation to new environments
Indeed, our upright walking life style gave our hands a new freedom that allowed us homonids to make and use tools and weapons. This stimulated rapid brain development that eventually gave rise to the evolution of language and human consciousness, which is closely entwined with the evolution of technology and social relations (Capra and Luisi 2014).
4.3.1 Consciousness
With this evolution a second type of consciousness arose, which is referred to as extended consciousness (see e.g. Capra and Luisi (2014)). So on the one hand we have primary consciousness that is the cognitive process that is accompanied by basic perceptual, sensory and emotional experience, which gives an organism a sense of self here and now, and, is widespread among living organisms. On the other hand, extended consciousness involves a more elaborate self-awareness, an experience of identity, the ability for reflection and to hold mental images, which eventually enabled us to formulate values, beliefs, goals and strategies. From the evolution of language not only an inner world of concepts and ideas emerged but also a social world with organised relationships and culture (Capra and Luisi 2014).
This organised social world has been key for our reproductive success. But, also came with profound consequences for our global ecosystem, which we changed in unprecedented ways. Our social organisation and human technology enabled us to make a giant jump from hominids who typically had a position in the middle of the food chain towards our position at the top of the food chain (Harari 2015). Other species at the top of the food chain evolved over a course of millions of years into that position, which enabled ecosystems to slowly adapt to them. We, humans, however, took this spectacular leap so quickly that the ecosystem and our own psycho-emotional system had no time to adjust. Indeed, top predators are majestic creatures full of confidence as they evolved over millions of years with almost no natural enemies, while mankind took that position so quickly that we are still confronted with the fears and anxieties that are associated to species with a position at the middle of the food chain (Harari 2015), which can have far reaching consequences on how we act and react. Therefore, it is key that we learn to connect to our numinous unconsciousness, to our greatness with humbleness, and, as such can evolve from exploiting the world towards respecting all the beauty that is surrounding us.
Similar to biology, our social culture also evolves, but at a much faster and ever increasing pace. Our current social culture, however, overemphasizes our extended consciousness, which led in extremis to the radical humanism of Decartes, which is summarized in his famous quote “je pense donc je suis”.
Our self, however, is a mental image, which is very nicely worded by Brad Blanton in his book “Radical Honesty”: “We are the Being, the source of being and remembering. The creator of our own universe. We awaken in the womb into the ocean of experiences. Over a long period of time, that ocean becomes a sea of suggestions. We lose track of the ocean of experiences. We lose track of having created the sea. After we have lost track of everything sufficiently, we continue to interact with the sea and create a self. What we call the self is a creation of further interactions of the sea of suggestion. The being we were when we began, the being we actually still are, alive in an ocean of experience, including all the ocean as itself, recedes to the background of our attention, and the self we have created comes to the foreground. As we identify with our newly created self, we lose touch with the being we are and have been since light came on.” (Blanton 1996).
This has also been confirmed in scientific experiments. Kahneman (2012), for instance, showed in his cold water experiments that we have an experiencing self, which refers to our primary consciousness that resides in the ocean of experiences, and, a remembering or narrative self associated with our extended consciousness that can create mental images and memories of our experiences, which both play an important role in our decision making. In these experiments he exposed people to two treatments. In one treatment, a person’s hand was immersed in cold water at 14 degrees for 1 minute. In the other treatment, he immersed their hand in water at 14 degrees for 1 minute, which is as annoying as the first treatment, and then the water temperature was raised to 15 degrees, which is only slightly less annoying, for an additional 30 seconds. Our experiencing self would never consider that extending the treatment with an episode of slightly warmer water makes the whole episode more appealing. But, when people were asked almost everyone preferred the longer treatment where the water is less cold at the end. Storing experiences happens according to a peak/end rule, i.e. according to the maximum intensity of the experience and its ending, and, it is our remembering self that makes decisions. The peak/end rule is a heuristic that evolved biologically and allows us to quickly and efficiently store experiences without the burden to remember every instant of the entire episode. This rule works very well in general, however, it can lead to peculiar choices in specific situations.
4.3.2 Thinking fast and slow
In his book Thinking, Fast and Slow Kahneman (2012) also discusses many other heuristics that lays at the heart of our decision-making. Indeed, he pointed out that we have two systems, a fast system and a slow system.
Fast thinking happens when facing problems that comes to mind automatically, e.g. when seeing a picture of an angry person we immediately know that they is angry. This way of thinking happens through our instincts, emotions, intuition and associative activation. Experiences and words evoke memories, which evoke emotions that in turn trigger physiological responses, facial expressions and other reactions. This all happens extremely quickly and all at once and results in a self-reinforcing pattern of integrated cognitive, emotional, physiological and physical responses.
Slow thinking involves mental work and happens when we face problems for which the solution does not come to mind or that do not seem trivial. It is strongly associated with our extended consciousness. Note, however, that Kahneman points out that our slow thinking is also embodied. Indeed, during the slow thinking process our muscles tense up, our blood pressure rises, our heart rate increases and our pupils dilate, among others. Although many humans experience themselves as independent individuals who make their decisions in a rational and well thought out way, Kahneman (2012) further argues that the majority of our decision making happens through fast thinking.
4.3.3 Implications for our well-being and Biodanza
It is thus important for our well-being to be well connected to our instincts and emotions. Indeed, as Rolando Toro pointed out, our instincts and emotions are the result of million of years of evolution. They are a shared cognition that has been differentiated, shaped to maintain and reproduce our species, and are the result of the environments and evolutionary development that our ancestors, i.e. humans and other species from which we evolved, underwent up to this point.
In our current society, however, there is a large emphasis on our extended consciousness. Moreover, information technology such as social media are also designed to hack our fast thinking process with the sole purpose to manipulate us for economic purposes. Together with the repression of our instincts and emotions this can invoke a dissociation between our cognitive processes, our bodily experiences and our needs, which in turn can lead to psycho-emotional pathologies.
With Biodanza we strengthen our identity and with exercises evoking biological regression we feed our primary consciousness, which lets us reconnect with our perceptual, sensory and emotional experiences, and, our instincts, here and now. This can restore our primary cognition that has evolved over the course of millions of years. The vivencia can reactivate vital physiological and psycho-emotional processes and can have a deep impact on our being. Moreover, in the reactivation phase of the session, we reactivate our extended consciousness and often color our mental images and memories with the deep vivencial experience.
Now that we introduced how all species could evolve from their last universal common ancestor and touched upon some specific evolutionary aspects of our human species, we are ready to make the transition to how we humans evolve in our life span. This is also referred to as ontology, which is a another crucial biological aspect in the Model of Biodanza and is our focus in the next chapter.
References
Phototaxis: movement and/or growth towards or away of light↩︎
Geotaxis: movement and/or growth in response to a gravitational field↩︎
Chemotaxis: movement and/or growth towards or away of a chemical or a biochemical concentration gradient↩︎
Gamete: sperm or egg cell↩︎
Anaerobic: living in the absence of oxygen↩︎
Note, that according to Margulis the large anaerobic cell with a nucleus, the first eukaryote, was already the result of an earlier endosymbiotic event. However, there is no scientific consensus on this hypothesis, yet.↩︎
Aerobic: oxygen using and respiration with oxygen provides more energy↩︎
Large groups of a kingdom are more formally referred to as phyla↩︎
Section Misunderstandings about natural selection p20↩︎